Introduction
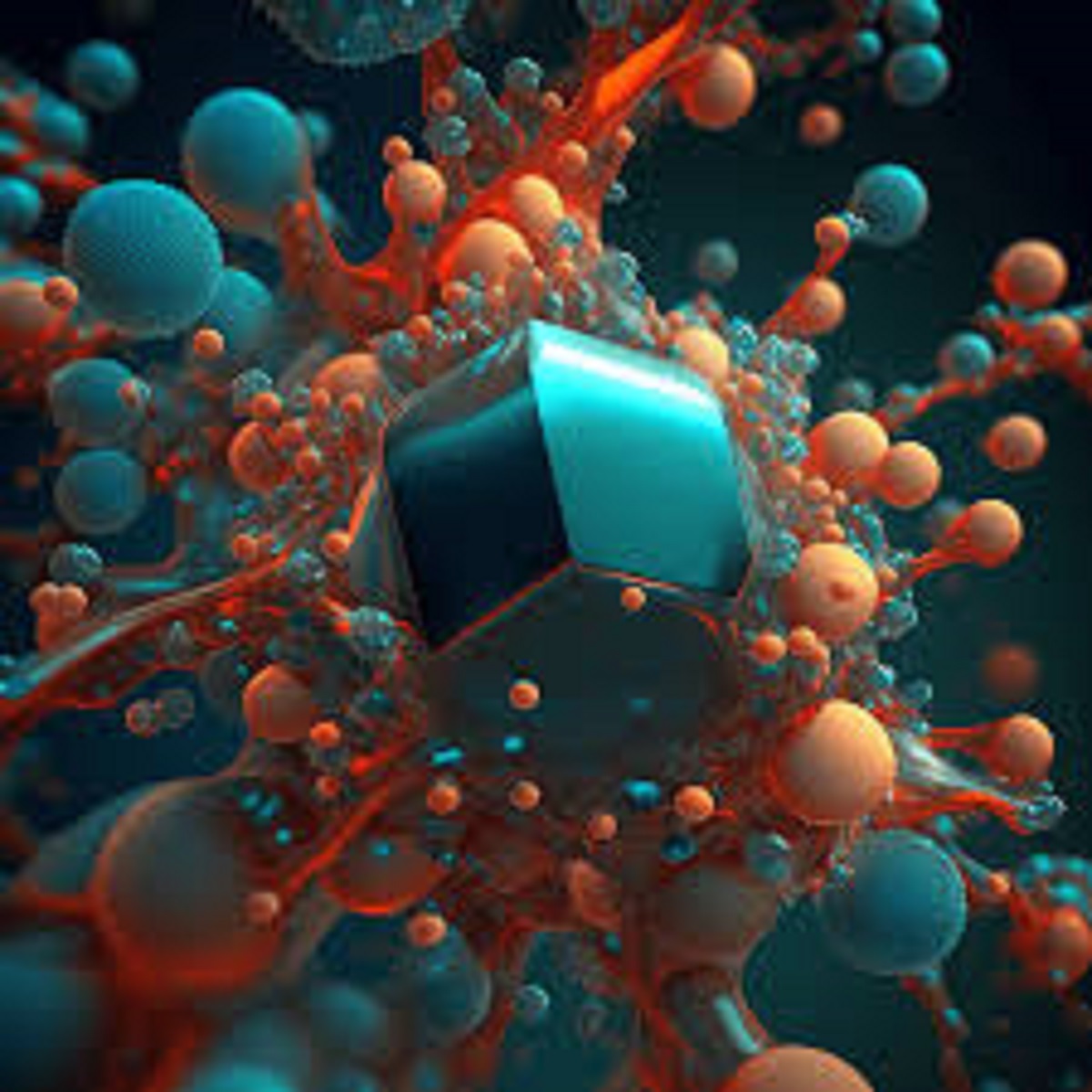
Atoms and molecules combine to form dynamic structures and systems that are the building blocks of every organism’s existence. For humans, cell membranes, hormones, and DNA are examples of vital structures that measure in the nanometer range. In fact, every living organism on earth exists because of the presence and interaction of various nanostructures. Nanotechnology deals with the capability to image, measure, model, control, and manipulate matter at dimensions of roughly 1–100 nanometers, where novel interfacial phenomena introduce new functionalities.
This exceptional capability has led to a vast array of new technologies that have an impact on virtually every aspect of science and technology, industry, economy, the environment and human lives.
All organisms represent a consolidation of various nanoscale-size objects. Even food molecules such as carbohydrates, proteins and fats are the results of nano scale-level mergers between sugars, amino acids, and fatty acids.
The electron microscope and, more recently, the development of tools such as probe microscopes, has provided unparalleled opportunities for understanding heterogeneous food structure at the sub-molecular level.
This has provided new solutions to previously intractable problems in food science and offers new approaches to the rational selection of raw materials, or the processing of such materials to enhance the quality of food products.
As it applies to the food industry, nanotechnology involves using biological molecules such as sugars or proteins as target recognition groups for nanostructures that could be used, for example, as biosensors on foods. Such biosensors could serve as detectors of food pathogens and other contaminants and as devices to track food products.
Nanotechnology may also be useful in encapsulation systems for protection against environmental factors. In addition, it can be used in the design of food ingredients such as flavors and antioxidants. The goal is to improve the functionality of such ingredients while minimizing their concentration.
The recent explosion in the general availability of nanoproducts makes it almost certain that nanotechnology will have both direct and indirect impacts on the food industry.
Some nanoscale phenomena have been utilized in nutraceutical and functional food formulation, manufacturing, and processes. New concepts based on nanotechnology are being explored to improve product functionality and delivery efficiency. Some of these nano-based technologies are outlined below.
Nanoparticulate Delivery Systems for Foods
Systems containing large interfacial areas such as emulsion, dispersion, and bi continuous structured fluid are a rich source of new knowledge.
Newly developed capabilities in nanoscale characterization offer a better visualization of these structures in nanometer resolution, and further a better understanding of their functionality.
When amphiphilic molecules like surfactants, lipids, and copolymers that have both polar and nonpolar characteristics are dispersed in a polar solvent, hydrophobic interactions cause them to spontaneously self-assemble into a rich array of thermodynamically stable, lyotropic, liquid crystalline phases with characteristic length scales in the nanometers.
These include micelles, hexagonal (tubular) structures, lamellar structures, and cubosomes, which possess a high degree of molecular orientation order despite the fact that they exist in a liquid state.
Micelles:
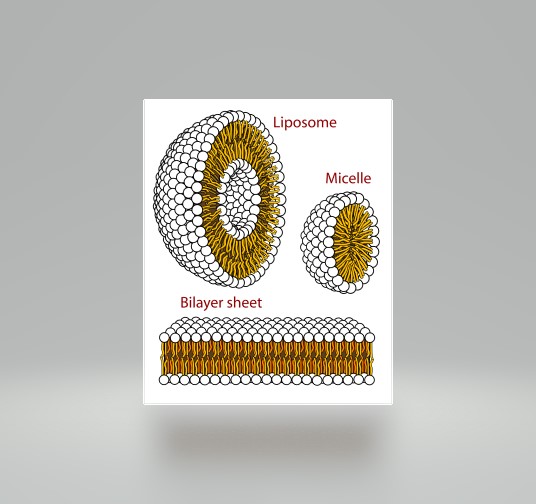
These are submicron spherical particles, typically 5–100 nm in diameter, that are formed spontaneously upon dissolution of surfactants in water at concentrations that exceed a critical level, known as the “critical micelle concentration” (CMC).
This self-assembly process is thermodynamically driven; i.e., interactions of the hydrophobic tail group of surfactants with water are minimized, while interactions of the hydrophilic surfactant head groups with water are maximized.
Because of this, micelle integrity under a given set of environmental conditions (pH, temperature, salt concentration) is often maintained for many years.
A remarkable property of micelles is that they have the ability to encapsulate nonpolar molecules such as lipids, flavorants, antimicrobials, antioxidants, and vitamins.
Compounds that ordinarily are not water soluble or are only sparingly soluble can, with the help of micelles, be made water soluble.
Micelles containing solubilized materials are referred to as microemulsions or swollen micelles. While micelles have been used as a delivery system for pharmaceutical compounds for quite a long time, their use as carrier systems for functional food components has only recently attracted increased attention.
Reports of successful application of microemulsions include encapsulation of limonene, lycopene, lutein, and omega-3 fatty acids using a variety of food grade emulsifiers, although in some cases addition of ethanol as a co-surfactant was required.
Liposomes:
Liposomes or lipid vesicles are formed from polar lipids that are available in abundance in nature, mainly phospholipids from soya and egg.
Like micelles, liposomes can incorporate a wide variety of functional components in their interior. However, in contrast to micelles, they can be used to encapsulate both water and lipid-soluble compounds.
Liposomes are spherical, polymolecular aggregates with a bilayer shell configuration. Depending on the method of preparation, lipid vesicles can be unilamellar or multilamellar, containing one or many bilayer shells, respectively.
Liposomes typically vary in size between 20 nm and a few hundred micrometers. Their core is aqueous in nature, its chemical composition corresponding to that of the aqueous solution in which the vesicles are prepared.
Because of the charge of the polar lipids used in the preparation of liposomes, charged but water-soluble ionic species can be trapped inside the liposomes. The pH and ionic strength of the liposomal core can thus differ from those of the continuous phase in which the liposomes are later dispersed.
Liposomes have been successfully used to encapsulate proteins and provide a microenvironment in which proteins can continue to function regardless of external environmental conditions. On the other hand, the interior of the bilayer has properties resembling those of an organic solvent.
Consequently, lipid compounds can be encapsulated inside the bilayer, a process known as adsolubilization.
Liposomes have been shown to increase shelf life of dairy products by encapsulating lactoferrin, a bacteriostatic glycoprotein as well as nisin Z, an antimicrobial polypeptide.
Antimicrobial efficiency of other ingredients in the encapsulated form has also been reported.
Liposomal entrapped phosvitin was used to inhibit lipid oxidation in a variety of dairy products and ground pork.
Recent research has demonstrated that, liposome encapsulated vitamin C retained 50% activity after 50 days of refrigerated storage, whereas free ascorbic acid lost all activity after 19 days.
Nanoemulsions:
These are simply very fine oil-in-water (o/w) emulsions with mean droplet diameter of 50–200 nm.
An emulsion is defined as a mixture of two completely or partially immiscible liquids, such as oil and water, with one liquid being dispersed in the other in the form of droplets.
Examples of emulsified food products are mayonnaise, milk, sauces, and salad dressings. In contrast to these well-known o/w emulsions, nanoemulsions are small enough not to scatter light in the visible region of the spectra; thus, they appear clear instead of being optically opaque.
Because of their small size, they also do not cream within an appreciable time. Creaming is the process whereby oil droplets move to the top of the emulsion to form a concentrated oil-droplet layer. This is often followed by a complete breakdown of the emulsion, yielding a clearly visible oil layer on top of the emulsion.
Nanoemulsions and macroemulsions can be manufactured in a similar fashion using high-pressure homogenizers, or membrane and microfluidic channels.
It should be noted that the proper choice of surfactants and/or polymers is critical in the production of nanoemulsions.
Because of their small size, nanoparticles have excellent penetration properties to ensure rapid delivery of high concentrations of active ingredients to cell membranes.
Bioavailability of lipophilic active ingredients can be substantially improved by delivery in Nanoemulsions.
For example, nanoemulsions have been used in parenteral nutrition for quite some time. Also because of their small size, they may also exhibit some interesting textural properties that differ from those of an emulsion containing larger droplets.
For example, they may behave like a viscous cream even at low oil droplet concentrations, a fact that has attracted attention in the development of low-fat products.
Biopolymeric nanoparticles:
These consist of a matrix of biopolymers that may be linked through intermolecular attractive forces or through chemical covalent bonds to form solid particles.
Nanoparticles may consist of a single biopolymer or may have a core-shell structure. Because of the versatility in terms of compounds that can be encapsulated and the degree to which these particles can be engineered and surface properties can be tailored, they have rapidly become the most promising nanoscale delivery systems in the pharmaceutical and cosmetics industries.
Food-grade biopolymers such as proteins or polysaccharides can be used to produce nanometer-sized particles. Using aggregative (net attraction) or segregative (net repulsion) interactions, a single biopolymer separates into smaller nanoparticles.
The nanoparticles can then be used to encapsulate functional ingredients and release them in response to distinct environmental triggers.
One of the most common components of many biodegradable biopolymeric nanoparticle is polylactic acid. But its high cost and susceptibility to hydrolytic breakdown were believed to make it unsuitable for use in biomedical or agricultural applications or sparingly used in research.
However, the use of this polymer as an ideal material for sutures was discovered in the 1970s, and a process was developed in the 1980s to produce the polymer via bacterial fermentation, greatly reducing costs and increasing production rates.
Today, a wide variety of natural and synthetic polymers have been used to encapsulate and deliver compounds. Among these are chitosan, a natural antimicrobial and anti-oxidative polymer obtained from crustacean shells and the synthetic polymers L-, D-, and D,L-polylactic acid (PLA), polyglycolic acid (PGA), and polycaprolactic acid (PCL).
Copolymers created using combinations of the monomers lactide, galactide, and caprolactone are also increasingly used.
Cubosomes:
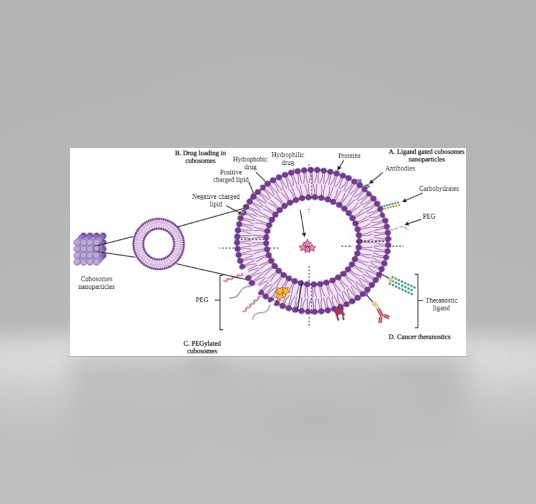
These are bicontinuous cubic phases which consist of two separate, continuous, but non-intersecting hydrophilic regions divided by a lipid layer that is contorted into a periodic minimal surface with zero average curvature.
The continuous and periodic structure results in a very high viscosity of the bulk cubic phase. However, cubosomes prepared in dispersion maintain a nanometer structure identical to that of the bulk cubic phase but yield a much lower, water-like viscosity.
Its tortuosity can be useful for slowing diffusion in controlled transport applications.
Its isotropic optical property permits uses in many different products. Compared to liposomes, cubosomes have much higher bilayer area-to-particle volume ratios. The cubosome structure can be changed by modifying the environmental conditions, such as pH, ionic strength, or temperature, thus achieving controlled release of the carried compound.
Cubosomes may be used in controlled release of solubilized bioactives in food matrices as a result of their nanoporous structure (approximately 5–10 nm); their ability to solubilize hydrophobic, hydrophilic, and amphiphilic molecules; and their biodegradability and digestibility by simple enzyme action.
The cubic phase is strongly bioadhesive, so it may find applications in flavor release via its mucosal deposition and delivery of effective compounds.
Yet, its tortuous structure may lead to applications where masking unpleasant taste or flavor is desirable, because of the slow effective diffusivity. The rate of release appears tunable through system optimization or ideal formulation of products for specific purposes.
Nanolaminates:
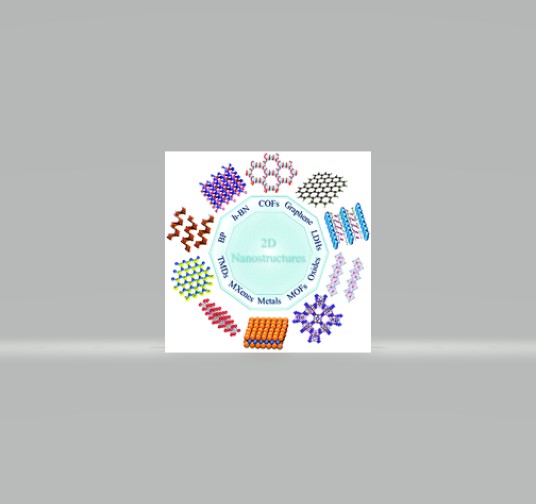
Besides nanodispersions and nanocapsules, another nanoscale technique that is commercially viable for the food industry is nanolaminates.
Consisting of two or more layers of material with nanometer dimensions, a nanolaminate is an extremely thin food-grade film (1–100 nm/ layer) that has physically bonded or chemically bonded dimensions.
Because of its advantages in the preparation of edible films, a nanolaminate has a number of important food-industry applications.
Edible films are present on a wide variety of foods: fruits, vegetables, meats, chocolate, candies, baked goods, and French fries.
Such films protect foods from moisture, lipids, and gases, or they can improve the textural properties of foods and serve as carriers of colors, flavors, antioxidants, nutrients, and antimicrobials.
Currently, edible nanolaminates are constructed from polysaccharides, proteins, and lipids.
Although polysaccharide- and protein-based films are good barriers against oxygen and carbon dioxide, they are poor at protecting against moisture.
On the other hand, lipid-based nanolaminates are good at protecting food from moisture, but they offer limited resistance to gases and have poor mechanical strength.
Because neither polysaccharides and proteins, nor lipids provide all of the desired properties in an edible coating, researchers are trying to identify additives that can improve them, such as polyols.
For now, coating foods with nanolaminates involves either dipping them into a series of solutions containing substances that would adsorb to a food’s surface or spraying substances onto the food surface. While there are various methods that can cause adsorption, it is commonly a result of an electrostatic attraction between substances that have opposite charges.
The degree of a substance’s adsorption depends on the nature of the food’s surface as well as the nature of the adsorbing substance.
Different adsorbing substances can constitute different layers of a nanolaminate like polyelectrolytes (proteins and polysaccharides), charged lipids, and colloidal particles.
Consequently, different nanolaminates could include various functional agents such as antimicrobials, anti-browning agents, antioxidants, enzymes, flavors, and colours.
Conclusion
Undoubtedly nanotechnology is having potential applications in all areas of food production and processing, however many of the methods are either too expensive or too impractical to implement on a commercial scale.
There is an urgent need for nanoscale techniques that are most cost-effective in development of new functional materials, food formulations, food processing at microscale and nanoscale levels, product development, and storage.
Although the products of nanotechnology intended for food consumption are likely to be classified as novel products, they require testing and clearance, and there are concerns, particularly in the area of food contact materials, that there could be inadvertent release and ingestion of nanoparticles of undetermined toxicity.
Such concerns need to be addressed because the ultimate success of products based on nanotechnology will depend on consumer acceptance.
Consideration should be given to the consequences of the use of nanotechnology to enhance the bioavailability of nutrients. This should consider the safety of the products, the consequences of enhanced or altered metabolism, and also the need for labelling, regulation and testing of health claims for such food supplements.